We know Cannabis sativa L., either in the form of Group I cannabis or Group III hemp, for its wide range of industrial and medicinal applications. Its popularity and public acceptance have increased, particularly as a natural alternative to pharmaceutical compounds. Unfortunately, stigma around the use of cannabis persists, which hinders scientists who want a deeper understanding of both the plant’s genetic and phytochemical levels in the context of cannabis cultivation, agricultural research, and sustainable farming solutions.
Cannabis has enormous medicinal potential and is fertile ground for the discovery of novel compounds. An increasing involvement of traditional scientists in cannabis research adds legitimacy to this new frontier of medicine, helping to slowly erode the negative societal perceptions of cannabis.
There are several new advancements and recent applications of biotechnological methodologies for the genetic engineering and transformation of cannabis. These include:
- genetic manipulation to alter chemical expression, crucial for cannabis cultivation and agricultural research,
- the application of recombinant DNA technologies,
- the use of advanced analytical chemistry for the discovery of novel molecules
- the assessment of how environmental factors influence epigenetics and gene expression, a key aspect of sustainable farming solutions.
The cannabis industry can use these tools to produce more consistent and efficacious strains, while also contributing to the potential discovery of novel phytochemicals and promoting sustainable farming solutions. In the near future, these technologies will fill any of the gaps in the genetic and phytochemical variability that currently exist with traditional breeding techniques, thereby enhancing the scope of cannabis cultivation and agricultural research.
Genetic Manipulation
Current breakthroughs in genetic engineering are now enabling new approaches to improving cannabis cultivation for a more favorable outcome. As a result of these advancements, phytochemical quality and overall product efficacy can be significantly improved, as well as enhancing crop yields and resilience to pests, viruses, and any other environmental stressors.
Of these revolutionary techniques is the CRISPR/Cas system (clustered regularly interspaced short palindromic repeat), which has demonstrated the ability to remove and/or add particular genetic traits of interest with high efficiency and low cost. With CRISPR it is possible to produce ultra-premium cannabis/hemp with specific phytochemical characteristics to cater to individual needs — a tailor-made experience for the medical or recreational users.
Contrary to popular belief, the CRISPR-Cas9 tool was not created in the laboratory. It is, in fact, a natural reoccurring defense mechanism presented in bacteria that is responsible for recognizing viral DNA sequences and cutting them to destroy the infectious agent. This highly specific technology is now being exploited to cut out undesirable traits out of an organism’s DNA like a molecular pair of scissors.
This system’s ability to “knock out” specific genes means one can be completely removed prior to expression. Perhaps you don’t want any cannabis that smells like lavender. With CRISPR, you can remove the genes that encode the enzymes that produce this fragrant terpene with relative ease. Conversely, you could “knock in” or introduce a new gene, for example a green fluorescent protein (derived from jellyfish), to make a novelty strain that glows under specific wavelengths of light.
A more practical application of this technology could be targeted towards improved management of the prominent pathogen Hop Latent Viroid (HLVd), which we first came to know as “dudding disease.” Cannabis is the closest genetic relative to hops, where this virus originates from, and its effects can vary from no detectable symptoms to a catastrophic decline in plant growth and health. HLVd is a formidable challenge for growers and has caused considerable financial damage, as well as a lot of frustration with lackluster crop performance. CRISPR-Cas9 could edit the genes that encode for the receptors that HLVd targets for cellular entry, thus removing the lock for HLVd’s key and ultimately providing immunity.
Producing Rare Cannabinoids
Recombinant DNA technologies are not new, but they are now making their way into the cannabis industry. Scientists can use bacteria or yeast to make large amounts of the enzymes that are involved in producing cannabinoids. By making lots of these, scientists can boost the production of rare or minor cannabinoids that normally only occur in small amounts. This allows them to study and use some of the less common cannabinoids more easily.
We are using this technique in ongoing research in our lab at the University of Texas at El Paso, using Pichia pastoris yeast cells to produce the enzyme cannabichromic acid synthase (CBCAS). This particular enzyme converts cannabigerolic acid (CBGA, the precursor molecule to numerous other cannabinoids) into cannabichromic acid (CBCA), which is then converted into cannabichromene (CBC) after decarboxylation (Figure 1).
Figure 1. The biosynthetic pathways of THCA, CBDA, and CBCA are catalyzed by three synthase enzymes that act upon CBGA as a precursor. Commercially available cannabis and hemp genetics can exceed 30% w/w for THCA and CBDA, respectively; however, CBCA concentrations very rarely exceed 1% w/w. As such, we believe that the use of recombinant DNA technologies provides the cannabis industry with the greatest chance to produce large amounts of CBCA/CBC. The abbreviation “w/w” means that for every 100 grams of the total weight, 30 grams of it is the component in question. The remaining 70 grams would be other components.
Why do we need yeast cells to produce this enzyme when the cannabis plant already does this for us naturally? Different varieties of cannabis tend to make a lot of THCA and CBDA, which turn into THC and CBD, but they don’t make much CBCA, which turns into CBC. It’s common to find cannabis flowers with 30% THC or CBD, but only 1% or less CBC.
THC helps the cannabis plant absorb UV light and survive. But it’s unclear what purpose CBC serves for the plant. It may be that high levels of CBC, or the CBCAS enzyme that makes it, could actually harm the plant.
Since there’s so little CBC in regular cannabis, scientists want to use yeast cells to produce ample amounts of the CBCAS enzyme. They can then introduce the CBCAS to cannabis plants that naturally produce the precursor enzyme CBGA — the raw material that gets turned into THC, CBD, or CBC. By giving the plants extra CBCAS enzyme, they can possibly produce higher amounts of CBC cost effectively. This would enable scientists to study CBC more and use it the same way they already use THC and CBD.
Unfortunately, it is currently unclear whether the removal of one enzyme would result in a simultaneous increase of others, or if the removal of a gene like CBDAS would have lethal consequences to the plant. In addition to making cannabinoids like CBD, enzymes like CBDAS probably also have other important jobs in the organism. It is important to remember that mother nature generally does a pretty remarkable job, and that any form of genetic modification or manipulation can have unintended consequences.
With that being said, using recombinant DNA technology is probably a better way to increase CBCA and CBC levels in cannabis and hemp, rather than genetically modifying the plants themselves. In this case, scientists could insert the cannabis genes for making CBCA into bacterial cells. Then they can grow huge batches of the bacteria and have them churn out the enzyme that produces CBCA. This can generate a lot of CBCA without needing to alter the actual cannabis genome. The ability to efficiently and affordably produce more amounts of CBCA using these cell cultures is important because CBC has been shown to protect nerve cells. There is also increasing anecdotal evidence that CBCA and CBC could be used to treat depression, and help prevent or delay the onset of neurodegenerative diseases such as Alzheimer’s. However, the low natural levels of CBCA in cannabis makes this a significant challenge.
Phytochemical Discovery
The discovery of new phytochemicals produced by cannabis represents one of greatest opportunities for social impact in this new frontier of medicine. Once you understand what the newly discovered molecules are, you can begin to explore how they affect the body, then possibly make chemical derivatives to treat conditions (Figure 2). The possibilities for new innovations are literally endless in cannabis, akin to the initial discoveries made with poppies in the 1900s.
Figure 2. Using advanced analytical instrumentation, we can possibly discover novel compounds. We can then characterize their functions using gene expression analyses (far right). This knowledge could ultimately lead to chemical derivatives that work even better than the original.
In addition to drug discovery, the use of advanced analytical instrumentation also has the potential for improving strain nomenclature and chemotyping. Breeding cannabis plants over many generations has made them less genetically diverse. There are thousands of different strain names, but many are just new versions of existing strains. Also, strains can be mislabeled or have inconsistent chemical makeup. This makes it hard to get a consistent product, therefore better analytical tools are needed to understand the different chemicals in cannabis plants, especially new ones we discover. These tools can also help classify cannabis strains based on their content of cannabinoids, terpenes, and flavonoids.
At the very least, a classification system should organize strains according to their levels of key chemicals. This will allow more targeted use by both patients and recreational users alike. More genetic diversity and chemical consistency is needed in cannabis strains and, hopefully, advanced analysis methods can help achieve this by detecting diversity and ensuring accurate labeling.
Environment and Expression
When cultivating cannabis, factors such as soil, water, nutrients, and lighting greatly affect the quality. Different strains react differently to environmental stresses, which can change their chemical contents and how their genes are expressed. It is critical to keep studying how the environment influences the phytochemicals like cannabinoids and terpenes in cannabis. The treatment of seeds and mother plants can significantly impact multiple generations of plants. For instance, the choice of lighting, such as starting seeds under a T4 bulb and later transitioning them to a full-spectrum LED for cloning, may have implications for subsequent clones, as well as any pollen or seeds they produce (Figure 3). The absence of full spectrum photons (400-700nm) from the T4 bulb could potentially result in downstream effects. Although this phenomenon is not yet fully understood, utilizing tissue culture to propagate clones might offer a solution to mitigate any negative impacts caused by accumulated poor treatment or “epigenetic baggage.”
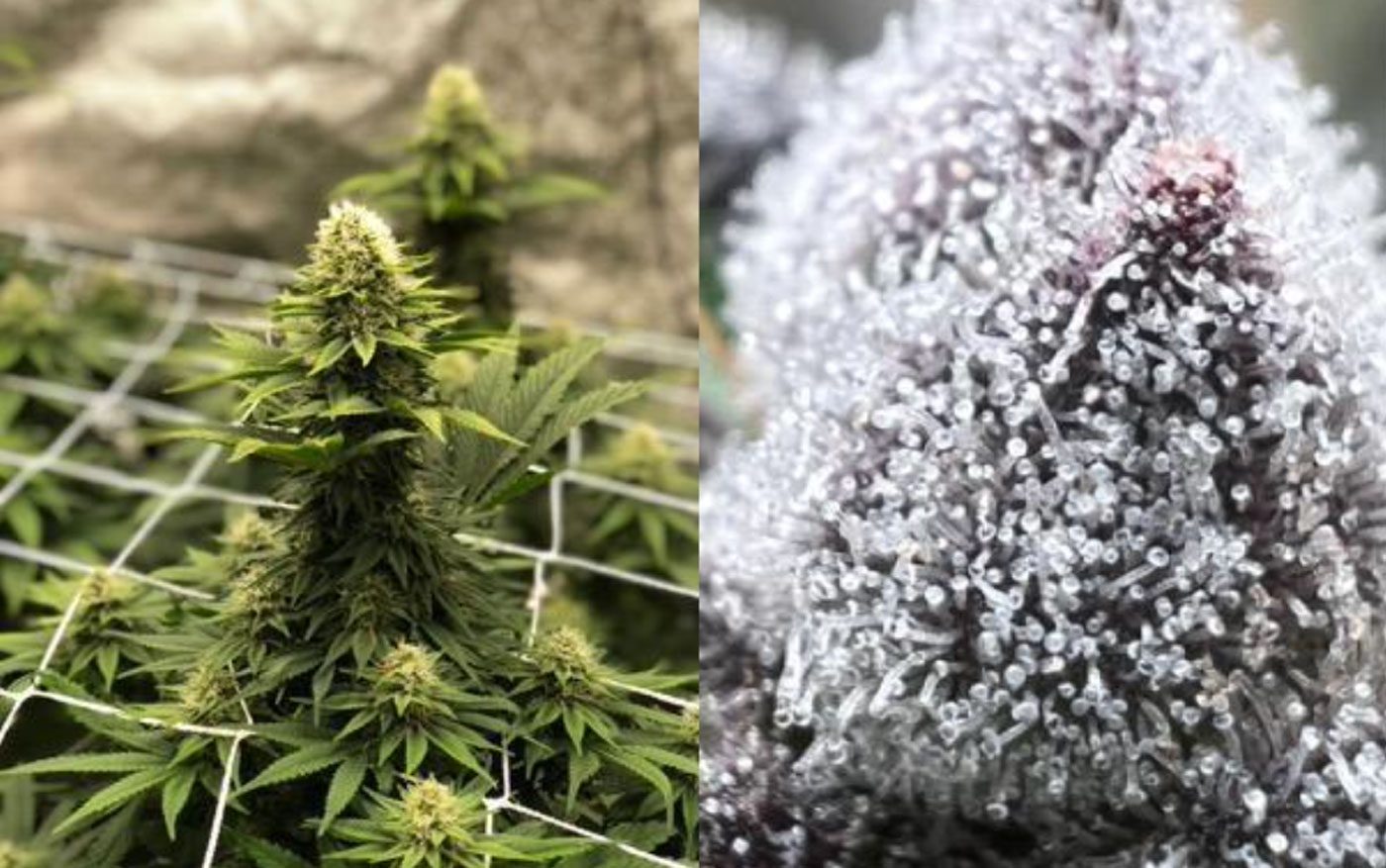
Figure 3. Lighting is the most influential variable in any cultivation environment, and is likely the largest driver of epigenetic change. The combination of different genetics with different lighting regimes can have a profound impact on phytochemical content, as well as likely influencing phytochemical expression and trichome density in subsequent generations. On the left, high-CBG genetic grown by Clean Gardens in Vaduz, Liechtenstein. On the right, high-THC genetic grown by OK Skunkworks in Oklahoma. Genetics by Agsense and lighting by Curtis Mathes Grow Lights.
The Bottom Line
The new era for personalized medicine is approaching, with cannabis cultivation leading the way in agricultural research and sustainable farming solutions. We can figure out which cannabis compounds could possibly alleviate and target specific symptoms within an individual. Tools that have been in use in other fields of medicine could soon help researchers in the cannabis cultivation and agricultural research sectors obtain high-quality cannabis with the perfect phytochemical profile for a specific health condition. Whatever the future holds, it is nice to know that there is a growing arsenal of tools that growers can use to dial in their products and continue innovating in sustainable farming solutions.